The American Physical Society’s (APS) March meeting takes place this week (14-18), drawing international leaders in physics from industry, academia, and private research. The Pritzker School of Molecular Engineering (PME) and the University of Chicago will have strong representation at this year’s meeting:
- 2 PME faculty honored—Prof. Giulia Galli and Prof. Liang Jiang
- 9 Invited speakers from the Pritzker School of Molecular Engineering
- 22 Invited speakers from the University of Chicago
- Quantum open house
The APS meeting is a leading venue for presenting the scientific advances that are the basis for novel and improved high-tech devices, imaging instrumentation, and materials. More than 10,000 technical papers will be presented at the meeting, covering a broad spectrum of topics, including beams physics, complex fluids, lattice properties, magnetism, nonlinear phenomenon, quantum computing, quantum metrology, spintronics, and superconducting materials.
Awards and recognition
Two professors from Pritzker Molecular Engineering will be accepting major awards during the meeting for their contributions to computational physics and quantum physics.
Giulia Galli, Liew Family Professor of Molecular Engineering, will accept the 2022 Aneesur Rahman Prize for Computational Physics. The Aneesur Rahman Prize is given annually and recognizes outstanding achievements in computational physics research. It consists of a $10,000 award and a certificate citing her contributions.
“It is, of course, a great honor for me to receive this award,” Galli said. “And many thanks go to the great work of my students, postdocs, and collaborators during the years and also to the support of my experimental colleagues, especially here at the University of Chicago.”
Galli will deliver the Rahman Lecture, “The universal language of quantum simulations,” during session G43: DCOMP Award Symposium.
Liang Jiang, professor of molecular engineering, received the 2022 Rolf Landauer and Charles H. Bennett Award in Quantum Computing. The award recognizes recent outstanding contributions in quantum information science, especially using quantum effects to perform computational and information-management tasks that would be impossible or infeasible by purely classical means. The award consists of a $5,000 prize and a certificate citing Jiang’s contributions.
“I am deeply honored to receive the Landauer-Bennett Award,” Liang said. “My work on quantum information is a highly collaborative research effort, and I am very grateful to all my collaborators. It is an exciting time to explore novel quantum phenomena. I am looking forward to working together with other researchers to tackle outstanding challenges so that we can fully harness the power of quantum information.”
Jiang will deliver his lecture, “Bosonic Quantum Error Correction,” during session K28: Landauer-Bennett Prize Award Symposium.
Quantum open house
The Chicago Quantum Exchange (CQE) organized several offerings during the March APS meeting, beginning with a quantum open house on March 13.
The open house, held in the Prairie Room from 4:00-7:00 PM, invited all educators and members of the community to learn about quantum education efforts and workforce development programs. The event offered quantum curricula for teachers, information for professionals, quantum games, and a panel discussion from 4:00-4:30 PM on integrating QISE into the undergraduate curriculum.
PME speaker highlights
Creating and controlling spin qubits through molecular engineering

David D Awschalom, Liew Family Professor of Molecular Engineering and Vice Dean for Research and Infrastructure, the Pritzker School of Molecular Engineering; Senior Scientist, Argonne National Laboratory; Director of the Chicago Quantum Exchange
Tuesday, March 15, 11:30 AM –12:06 PM (session time 11:30 AM–2:30 PM)
Room W-175C
Spin-based defects within semiconductors are used to construct quantum devices and machines that enable information processing and sensing technologies based on the quantum nature of electrons and atomic nuclei. These materials possess an electronic spin state that can be employed as a quantum bit over a range of temperatures. They have a built-in optical interface in the visible and telecom bands, retain their quantum properties over millisecond timescales or longer, and can be manipulated using a simple combination of light and microwaves.
Alternatively, molecular spin systems are attractive building blocks for quantum information science. Through a chemical approach, bottom-up design of qubits enables atomistic tunability of their environment, scalability to multi-qubit architectures using directed assembly, and portability between various host materials and devices. With these functionalities-which are challenging to achieve with semiconductor defects-designer qubits could be synthesized for a diverse range of applications from quantum sensing in biosystems to the creation of nodes in a quantum network.
To demonstrate that the enabling features of solid-state defects can be translated into a molecular architecture, we discuss transition metal-based molecular spins with optical addressability. These molecules comprise a central chromium ion coordinated to surrounding ligands, enabling optical initialization and read out, as well as coherent microwave manipulation of the ground-state spin. We also show atomistic tunability of qubit properties by comparing molecules which differ by the placement of a single methyl group on the coordinating ligands, resulting in marked changes in emission and ground state energies, highlighting how molecular qubits can be tailored for specific applications. Overall, this work indicates the potential of a chemical approach for quantum engineering with optically addressable spin systems.
Rahman Prize (2022): The universal language of quantum simulations

Giulia Galli, Liew Family Professor of Molecular Engineering; professor of chemistry at the University of Chicago; senior scientist at Argonne National Laboratory; and director of the Midwest Integrated Center for Computational Materials (MICCoM),
Tuesday, March 15, 11:30 AM–12:06 PM (session time 11:30 AM–2:30 PM)
Room W-375B
We discuss studies of materials for sustainable energy sources and quantum technologies carried out using ab initio simulations. We show that, despite several approximations to the basic equations of quantum mechanics and the computational challenges in describing realistic size and time scales, insightful predictions can be made that are not only corroborated by experiments but inspire new ones.
Quantum error correction in quantum metrology

Sisi Zhou, graduate student
Tuesday, March 15, 12:06 PM–12:42 PM (session time 11:30 AM–2:30 PM)
Quantum metrology, which studies parameter estimation in quantum systems, has many important applications in science and technology, ranging from frequency spectroscopy to gravitational wave detection. Quantum mechanics imposes a fundamental limit on the estimation precision, called the Heisenberg limit, which is achievable in noiseless quantum systems, but is in general not achievable in noisy systems. This talk is a summary of some recent works on quantum metrology enhanced by quantum error correction. We present a necessary and sufficient condition for achieving the Heisenberg limit in noisy quantum systems. When the condition is satisfied, the Heisenberg limit is recovered by a quantum error correction protocol which corrects all noises while maintaining the signal; when it is violated, we show the estimation limit still in general has a constant factor improvement over classical strategies and is achievable using approximate quantum error correction. Both error correction protocols can be optimized using semidefinite programs. Examples in some typical noisy systems will be provided.
Landauer-Bennett Award (2022): Bosonic Quantum Error Correction
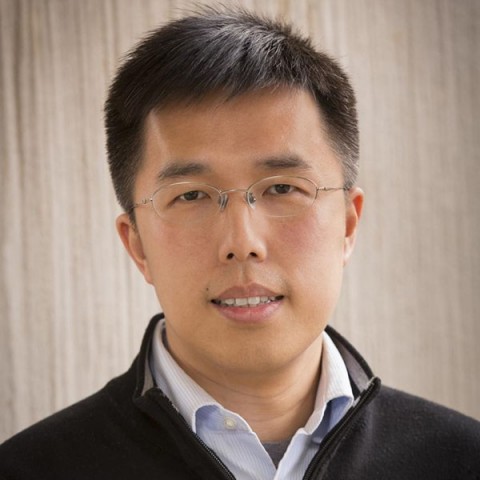
Liang Jiang, professor of molecular engineering
Tuesday, March 15, 4:48 PM–5:24 PM (session time 3:00 PM–6:00 PM)
Room W-190A
Bosonic modes are widely used for quantum communication and information processing. Recent developments in superconducting circuits enable us to control bosonic microwave cavity modes and implement arbitrary operations allowed by quantum mechanics, such as quantum error correction against excitation loss errors. I will discuss different bosonic encoding and error correction protocols and provide a perspective on using quantum error correction for various applications, including quantum computing, communication, simulation, and sensing.
Phonons as transporters of quantum information

Andrew Cleland, John A. MacLean Sr. Professor of Molecular Engineering Innovation and Enterprise, the Pritzker School of Molecular Engineering; Director, Pritzker Nanofabrication Facility
Wednesday, March 16, 12:42 PM–1:18 PM (session time 11:30 AM–2:30 PM)
Room W-375D
Phonons, and in particular surface acoustic wave phonons, have been proposed as a means to coherently couple distant solid-state quantum systems. Recent experiments have shown that superconducting qubits can control and detect individual phonons in a resonant structure, enabling the coherent generation and measurement of complex stationary phonon states. Here, I will describe our recent experiments demonstrating the deterministic emission and capture of itinerant surface acoustic wave phonons, enabling the phonon-mediated transfer of quantum states between two qubits, including the high-fidelity quantum entanglement of two superconducting qubits. If time permits, I will also describe a follow-on experiment that shows the phonon version of the optical “quantum eraser” experiment.
Stabilizing two-qubit remote entanglement with engineered synthetic squeezing

Aashish Clerk, professor of molecular engineering, the Pritzker School of Molecular Engineering
Wednesday, March 16, 1:18 PM–1:30 PM (session time 11:30 AM–2:30 PM)
Room W-196C
It is well known that qubits immersed in a squeezed vacuum environment exhibit many exotic phenomena, including dissipative entanglement stabilization. Here, we show that these effects only require interference between excitation and decay processes and can be faithfully realized without using non-classical light; instead, one uses simple classical temporal modulation. We present and analyze two schemes that harnesses this idea to stabilize entanglement between two remote qubits coupled via a transmission line or waveguide, where either the qubit-waveguide coupling is modulated, or the qubits are directly driven. Our protocols are especially well suited to state of the art circuit cavity QED systems featuring tuneable coupling elements, as well as more general circuit waveguide QED systems.
Five-second coherence of a single spin with single-shot readout in silicon carbide

Elena O Glen, graduate student
Wednesday, March 16, 3:36 PM–4:12 PM (session time 3:00 PM–6:00 PM)
Room Hyatt Regency Hotel -Jackson Park C
The neutral divacancy (VV0) in silicon carbide (SiC) is a spin defect that boasts a near-infrared spin-photon interface, long coherence times, and is hosted in a material that provides opportunities for wafer-scale semiconductor device engineering. Despite these advantages, single-shot readout (SSR)--deterministic measurement of the quantum state--has long been an outstanding hurdle for defect spins in SiC. Here, we present a demonstration of SSR of a single VV0 using spin-to-charge conversion (SCC). This SCC technique maps the spin state onto a long-lived charge state that can be optically read out. Using this technique, we achieve over 80% readout fidelity of the spin state without pre- or post-selection and harness the high signal-to-noise ratio of the readout to measure ultralong spin coherence times. We apply pulsed dynamical decoupling sequences to an isotopically purified host material and use SCC to measure single-spin T2 times exceeding five seconds, over two orders of magnitude greater than previously reported for this system. The SSR demonstrated here unlocks key capabilities for SiC-based systems, such as entanglement distribution and enhanced ac-sensitivity in quantum sensing schemes. These results also pave the way for integration of defects into single-charge sensitive, classical electrical devices.
Linking Intermolecular Geometry and Spin Coupling of Excitons in Organic Semiconductors

Leah R Weiss, postdoctoral scholar
Friday, March 18, 8:00 AM–8:36 AM (session time 8:00 AM–11:00 AM)
Room W-475B
The design and optimization of synthetic magnetic and optoelectronic materials requires precise chemical control of spin and electronic coupling. Here we focus on understandingthis structure-function relationship for light-harvesting applications of singlet fission: the production of two triplet excitons (each with spin S=1) following excitation of one singlet exciton (spin S=0). To quantitatively extract dipolar and exchange interactions between triplet excitons formed by singlet fission in a solid-state organic semiconductor, we have deployed broadband optically detected magnetic resonance, electron spin resonance, and magneto-optical spectroscopy. Mapping the experimentally extracted spin parameters onto the molecular crystal structure provides a window into exciton localization and coupling in the molecular lattice. This mapping of spin properties to excited-state electronic structure is made possible by sustained excited-state spin polarization and coherence over microsecond timescales. These results --linking intermolecular geometry and spin interactions-- provide a key step toward chemically controlling intermolecular spin coupling for both optoelectronic applications and molecular quantum technologies.
Polymer design in the era of machine learning
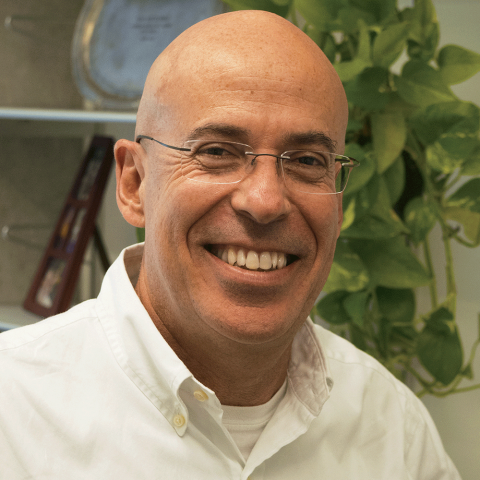
Juan de Pablo, Liew Family Professor of Molecular Engineering; Executive Vice President for Science, Innovation, National Laboratories and Global Initiatives at the University of Chicago
Friday, March 18, 1:18 PM–1:54 PM (session time 11:30 AM–2:18 PM)
Room W-184D
Advances in molecular modeling algorithms, optimization strategies, and machine learning techniques, are rapidly changing the way in which computational tools can be used to design polymeric materials systems or to interpret experimental data. In this presentation I will discuss these ideas by relying on three examples taken from our own research. In the first, I will discuss the automated creation of data bases, and the development of new graph based neural network strategies to represent polymeric structures. I will also discuss how such a framework can then be used to predict the properties of polymers and to design new materials with target properties. In the second, I will discuss how multiscale modeling and machine learning can be used to engineer the structure and, ultimately, the rheology of polymeric materials. In a third example, I will discuss how machine learning can be used to learn or extract physical principles from large data sets, particularly in the context of long-time structural relaxation in ordered polymeric materials.