Scientists have examined almost every corner of the periodic table seeking new materials for the superconductors needed to build quantum computers, magnetic resonance imaging, and many other high-tech applications.
“The traditional way of engineering superconductors is just you take materials and cook them in an oven to change their chemical composition,” said UChicago Pritzker School of Molecular Engineering Asst. Prof. Shuolong Yang. “Hopefully, what you create will have a higher superconducting temperature through these chemistry methods.”
There have been many success stories, but also increasing complexities in the engineering. For three UChicago researchers, a provoking question emerged: Is there a way to engineer superconductors without changing their chemical compositions?
Buoyed by a transformative $1.5 million grant from the Gordon and Betty Moore Foundation, Yang, PME Asst. Prof. Tian Zhong and UChicago Physics and James Franck Institute member Prof. Peter Littlewood look to harness an energy source first described by Albert Einstein to create an entirely new kind of superconductor.

Their novel approach would use photonic crystal cavities to sensitively control superconductivity by changing the physical environment, not the chemical one.
“If this is realized, it will be a completely new way of engineering superconductors,” Yang said. “This project not only has remarkable application prospects, but if it's successful, it will be a significant advance in fundamental science.”
To create these game-changing superconductors, the team is turning to the ambient background photons first described in the work that won Einstein the Nobel Prize.
‘Sometimes, we say there is light in darkness.’
Superconductivity is achieved when electrical resistance and magnetic fields vanish from a solid object. This makes that solid a perfect material for, among other uses, high-speed computing.
The phenomenon was first explained in the 1950s by John Bardeen, Leon Cooper and John Schrieffer in a work that would go on to net them their own Nobel. An electron moving through a solid vibrates the ions, creating a small well. A second electron coming along “remembers” the path the first one took and pairs with it, side-stepping the resistance it would have encountered if it had found its own way.
“Now we've got many other kinds of superconductors, but they fundamentally depend on the same kind of principle,” said Littlewood, who provided much of the theoretical framework for the UChicago team. “Something runs around and leaves an imprint in the space around it, which then provides a trajectory for a second particle to follow. They chase each other because this memory is created in the environment. We're proposing to do that with photons.”
The source of the photons would come from photonic crystal cavities the team is working to create, essentially nanoscopically small boxes with reflective interiors. The mirrored insides would reflect and intensify the photons, which would then propel atomic vibrations through solids. As the vibrations move through the solid, they would create those “memories” for their fellow electrons to follow, turning the solid into a superconductor.
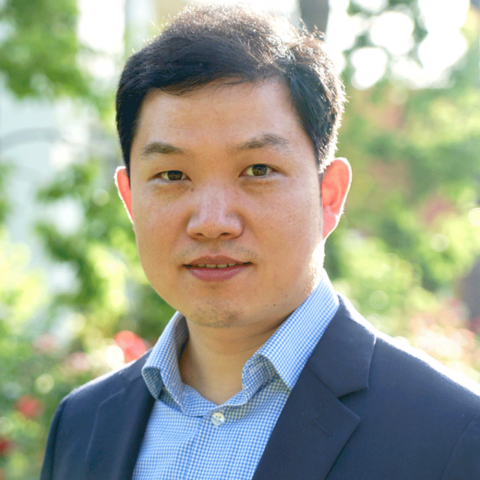
The team will not be adding photons into their cavities through a laser or any external light source. Instead, the cavities will reflect and intensify the existing background energy found in a vacuum.
“Sometimes, we say there is light in darkness,” said PME Asst. Prof. Tian Zhong. “In absolute darkness, there’s no light source, but there is actually a bit of photons and electromagnetic energy. That's what we are harnessing to create a superconductor.”
‘An entirely new way’
Creating the cavities themselves will be an interdisciplinary feat, combining Yang’s material science background and Zhong’s quantum photonics work.
“This will be an entirely new way to build superconductors, even though it is still far from large-scale production,” Yang said. “Instead of using chemistry methods, this is using quantum methods to engineer a quantum metabolic phenomenon.”
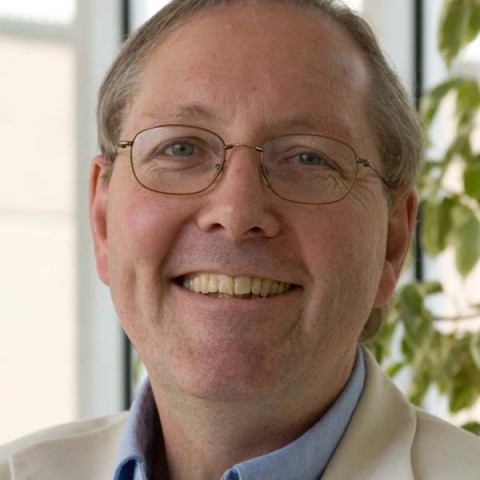
To make the cavities work, the wavelengths of the electrons and the photons from the vacuum should match.
Yang is working on lengthening the electron wavelengths, Zhong is working on shortening the photon wavelengths and Littlewood, in his words, is “trying to provide guidance about what would be the sweet spot.”
The $1.5 million Moore Foundation grant will help the team make this theoretically possible work a reality. The Gordon and Betty Moore Foundation advances scientific discovery, environmental conservation, and the special character of the San Francisco Bay Area.
Yang credits the Pritzker School of Molecular Engineering for its interdisciplinary approach that puts researchers from different areas side-by-side. He and Zhong first bandied the idea about during playdates for their children, before bringing Littlewood from UChicago Physics in to provide the theoretical framework.
“Without PME this would not have happened,” Yang said.